The balance between detrimental, pro-aging, often stochastic processes and counteracting homeostatic mechanisms largely
determines the progression of aging. There is substantial evidence suggesting that the endocannabinoid system (ECS) is part of the latter system because it modulates the physiological processes underlying aging1,2. The activity of the ECS declines during aging, as CB1 receptor expression and coupling to G proteins are reduced in the brain tissues of older animals3–5 and the levels of the major endocannabinoid 2-arachidonoylglycerol (2-AG) are lower6. However, a direct link between endocannabinoid tone and aging symptoms has not been demonstrated. Here we show that a low dose of D9-tetrahydrocannabinol (THC) reversed the age-related decline in cognitive performance of mice aged 12 and 18 months. This behavioral effect was accompanied by enhanced expression of synaptic marker proteins and increased hippocampal spine density. THC treatment restored hippocampal gene transcription patterns such that the expression profiles of THC-treated mice aged 12 months closely resembled those of THC-free animals aged 2 months. The transcriptional effects of THC were critically dependent on glutamatergic CB1 receptors and histone acetylation, as their inhibition blocked the beneficial effects of THC. Thus, restoration of CB1 signaling in old individuals could be an effective strategy to treat age-related cognitive impairments.
To determine whether prolonged exposure to a low dose of THC has lasting effects on learning and memory performance in mice aged 2, 12 and 18 months (termed young, mature and old, respectively), we implanted osmotic minipumps releasing 3 mg per kg bodyweight per day of THC or vehicle for 28 d. The effects of age and treatment on spatial learning and memory were first assessed in the Morris water maze (MWM) test starting on day 33 after pump implantation, thus allowing a washout period of 5 d (Fig. 1a). The mature and old vehicle-treated animals learned the task more slowly than the young animals, which is indicative of an age-related deficit in spatial learning (Fig. 1b). Treatment with THC improved task acquisition in mature and old animals. The difference between the groups persisted in the reversal phase of the test, which assessed learning flexibility. Thus, both THC-treated mature and old mice showed better performance than the vehicle-treated controls in the same age groups. In the probe trial phase of the test, which was conducted on day 6 before reversal learning and which is an indicator of long-term spatial memory, vehicle-treated old animals showed memory impairments, as indicated by reduced time spent in the target quadrant (Fig. 1c). Treatment with THC improved spatial memory in this age group to the level observed with the young controls. In young mice, THC treatment worsened performance, in good agreement with the known detrimental effects
of THC on cognition in young animals and humans7–9. Treatment with THC did not affect the time the mice required to reach a visible platform (Supplementary Fig. 1a).
We next used the novel object location recognition task. Young, vehicle-treated controls performed significantly better than vehicle-treated mature and old animals (Fig. 1d), which again indicates an age-related impairment of cognitive performance. THC treatment also abolished the cognitive deficit in mature and old animals in this test, as they performed at the same level as the young, vehicle-treated controls. THC treatment was already effective in mature animals after 14 d (Supplementary Fig. 1b). To further test the effect of THC treatment on long-term memory, animals were tested in the partner recognition test. Here the test mouse first explores an open-field arena with an unknown conspecific in a small grid cage and a small object.
This test was repeated after 24 h with a new partner in an identical grid cage instead of the object. If mice remember the previous partner from session 1, they will show a higher preference for the new partner. Mature and old animals did not remember the partner as well as young mice (Fig. 1e), but THC treatment restored partner-recognition ability in mature and old animals (Supplementary Fig. 1c). Together, these results reveal a profound, long-lasting improvement of cognitive performance resulting from a low dose of THC treatment in mature and old animals. THC treatment for 28 d restored the learning and memory performance of mature and old animals in the MWM, novel object location recognition and social
recognition tests to the levels observed in young mice.
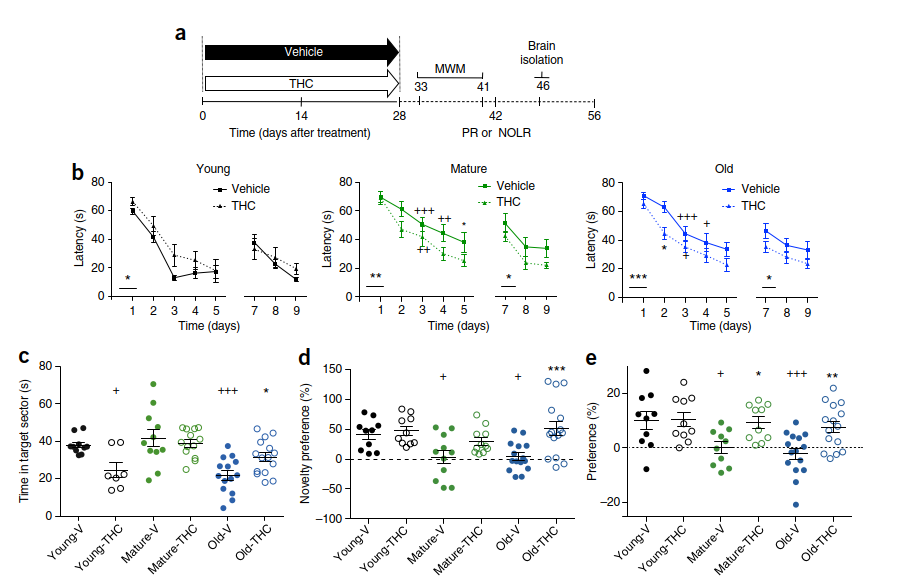
partner-recognition test. Preference for a new partner indicates partner recognition. The dashed line indicates the chance level. Young, n = 10; mature, n = 10; old, n = 15. *P < 0.05, **P < 0.01, ***P < 0.001, significant difference when compared to vehicle-treated mice; +P < 0.05, ++P < 0.01, +++P < 0.001, significant difference when compared to vehicle-treated young mice. Statistical significance was calculated using two-way ANOVA in b and one-way ANOVA in c–e, followed by Bonferroni’s t-test. In (c–e), circles represent data from individual animals. Means and s.e.m. are shown. See also Supplementary Figure 1 and Supplementary Table 4.
We next investigated the effect of THC on synaptic density in the hippocampus. Expression of the synaptic marker proteins synapsin I, synaptophysin and postsynaptic density protein 95 (PSD95) was lower in mature animals than in young ones (Fig. 2a), which is indicative of an age-related loss of synaptic connectivity10,11. THC treatment did not alter the expression of these proteins in young mice, but it increased the synapsin I and synaptophysin levels of mature animals to those observed in young, THC-free mice. These results were confirmed by immunohistochemistry (Supplementary Fig. 2). Further experiments
showed that THC increased hippocampal spine density and affected the balance of inhibitory versus excitatory synapses (Supplementary Results and Supplementary Fig. 2). We next established gene expression profiles in the whole hippocampus 50 d after minipump implantation. A total of 21,198 transcripts were identified. Hierarchical clustering of variably
expressed genes (P < 0.05, n = 2,090 genes) within the data set clearly revealed a four-group structure based on treatment and age (Fig. 2b and Supplementary Fig. 3). Most strikingly, THC-treated mature animals closely resembled vehicle-treated young mice, while THC treatment of young mice resulted in a gene expression pattern more similar to that of vehicle-treated mature animals. Using the two-way ANOVA model (P < 0.05, fold change = 1.5), we identified 100 transcripts differentially regulated by THC in young mice and 232 in mature mice (Supplementary Fig. 3). Of these, only 13 overlapped (Supplementary Fig. 4). Gene
ontology enrichment analysis (GOEA) followed by network visualization comparing the vehicle- and THC-treated old groups (Fig. 2c) identified large clusters of gene ontology (GO) terms associated with morphogenesis, homeostasis, gene expression, phosphorylation and nerve impulse transmission. This result indicates that THC treatment affects molecular processes relevant to cell plasticity and signaling in mature animals. Upregulated transcripts included Klotho (Kl) (Fig. 2d and Supplementary Figs. 5 and 6), which is known to extend lifespan in different species12–14 and to improve cognition15; transthyretin (Ttr), a gene that is thought to be protective against Alzheimer’s disease16,17; and brain-derived neurotrophic factor (Bdnf), an important neurotrophic factor that enhances synapse formation18 and cognitive functions19,20. The two transcripts that were most strongly downregulated in the mice after THC treatment corresponded to genes with potential pro-aging effects: caspase-1 (Casp1), which is involved in age-related impairments in cognition21, and connective tissue growth factor (Ctgf), which is known to enhance the pro-apoptotic activity of transforming growth factor β (TGF-β)22 (Supplementary Fig. 7). Together, these results demonstrate that the cognitive improvements in THC-treated mature mice were associated with a change in gene profiles; these changes and the associated cognitive improvements both lasted for several weeks after cessation of the treatment. The directions of the expression changes were such that the profiles of mature, THC-treated mice were most similar to those of young control mice, whereas THC treatment of young mice resulted in a gene expression pattern that was similar to that of vehicle-treated mature animals. This indicates that the enhanced CB1 tone achieved through low-dose THC treatment may have normalized the weak cannabinoid signaling signature in mature animals and thus reverted some of the age-related changes in gene expression, whereby several genes with antiaging effects were upregulated while genes contributing to aging were downregulated. The opposite transcriptional changes with THC treatment in young ani-
mals are interesting and deserve further investigation.
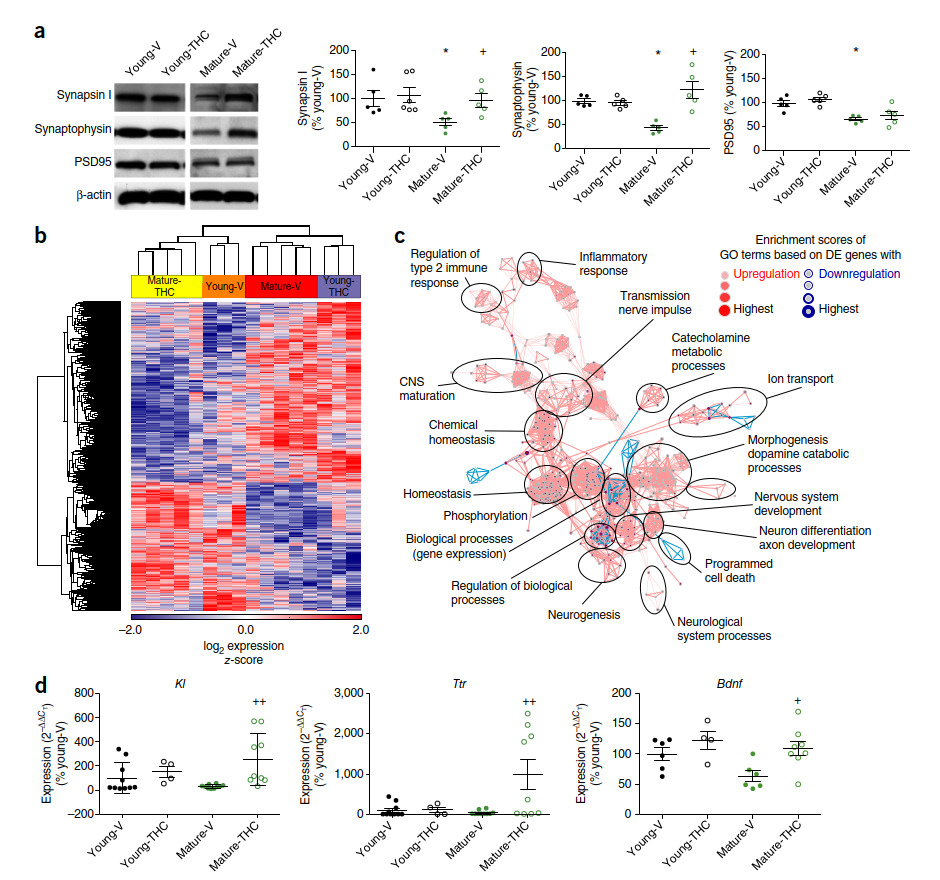
THC, similar to other drugs of abuse23, can activate cAMP-response-element-binding protein (CREB)24,25, a molecular switch that converts short-term, plastic changes into long-lasting adaptations26, thus playing a key role in learning and memory27. Increased CREB signaling is involved in the memory-promoting effect of young blood in models of parabiosis28 and caloric restriction29. THC can induce long-term changes in neuronal activity directly by the activation of genes with CREB-inducible promoter regions and indirectly through epigenetic changes induced by recruiting CREB-binding protein (CBP), a histone acetyltransferase that is specific for histones H3 and H4. To investigate whether these mechanisms contributed to the effects of chronic THC treatment, we analyzed CREB signaling, as well as the CB1-downstream Akt and extracellular-signal-regulated kinase (Erk) pathways. THC treatment significantly increased Erk1 and Erk2 (Erk1/2) and CREB phosphorylation in mature animals but not in young ones (Fig. 3a)30, whereas phosphorylation of Akt was not different between groups. Activated CREB
stimulates transcription of several genes that modulate synaptic plasticity, including Bdnf 31, and recruits the histone acetyltransferase CBP (p300)32. CBP facilitates acetylation of H3 and H4 histones, thus transforming chromatin into a relaxed configuration and making it more accessible to transcription factors33. The levels of acetylated H3K9 and H4K12 were significantly elevated (+60.0% and +22.9%, respectively) in the hippocampus of THC-treated mature animals in comparison to
control animals from the same age group, with these increases already visible after 14 d of THC treatment (Supplementary Fig. 8), whereas trimethylation of H3K9 was decreased (Fig. 3b). AcH2A, which is not regulated by CBP, remained unchanged after THC treatment. AcH3K9 was also markedly increased at the Kl promoter and at all four Bdnf promoters, as revealed by chromatin immunoprecipitation (ChIP) analysis (Fig. 3c). This may suggest that the increased expression of Bdnf and possibly other CBP-regulated genes is caused by THC-induced epigenetic changes. BDNF is an upstream activator and Bdnf is a major
downstream target of CREB-mediated signaling34. Expression of Bdnf and Ntrk2 (TrkB) decreases during aging35. Our results are consistent with such a CBP-mediated mechanism and with the demonstration that histone acetylation promotes cognitive functions, whereas the opposing process, histone deacetylation, negatively affects cognition36.
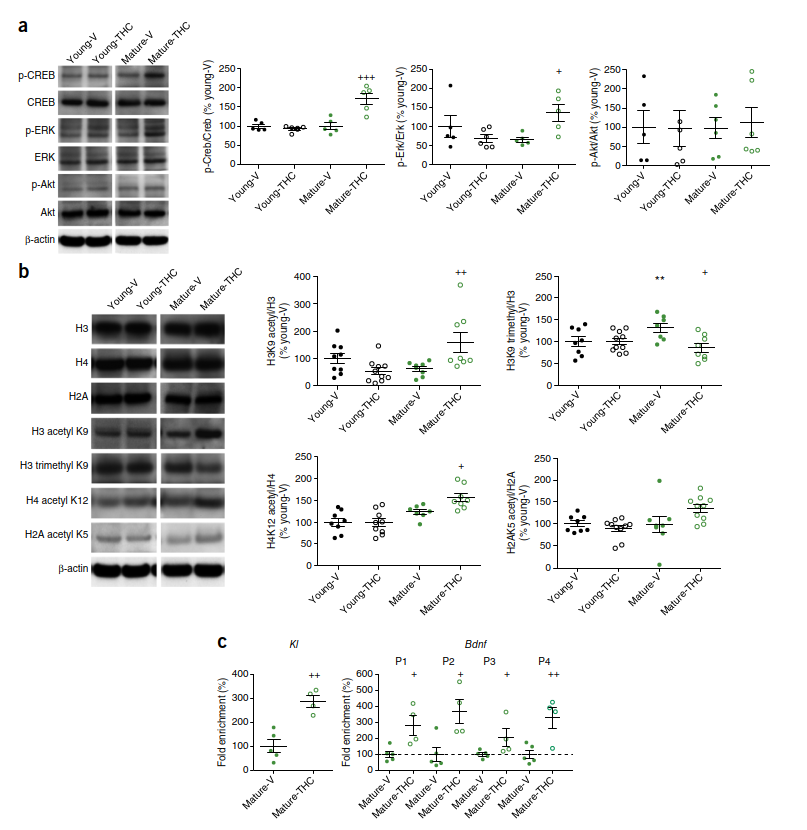
THC, n = 8; young-THC, n = 10; H3 trimethyl K9/H3: young-V and mature-THC, n = 8; mature-V, n = 7; young-THC, n = 10; H4 acetyl K12/H4: young-V and mature-THC, n = 8; mature-V, n = 7; young-THC, n = 9; H2A acetyl K5/H2A: young-V and mature-V, n = 8; mature-THC and young-THC, n = 10. (c) AcH3K9 enrichment was analyzed at the promoter regions of Kl and Bdnf by ChIP assays in the hippocampus of mature-V (n = 5) and mature-THC (n = 4) mice. *P < 0.05, **P < 0.01, difference between young-V and mature-V; +P < 0.05, ++P < 0.01, +++P < 0.001, difference between mature-V and mature-THC. One-way ANOVA followed by Bonferroni’s t-test was used to calculate significance in immunoblotting, and Student’s t-test was used for the ChIP analysis. Circles represent data from individual mice. P1, P2, P3,and P4 denote the four promoter regions of Bdnf. Vehicle controls were adjusted to 100%, as indicated by the dashed line. Means and s.e.m. are shown. See also Supplementary Figure 8, Supplementary Data, and Supplementary Table 3.
To directly test this hypothesis, a histone acetyltransferase inhibitor, anacardic acid (AA), was administered intraperitoneally on a daily basis to a new cohort of mature mice carrying osmotic minipumps releasing either vehicle or THC. AA treatment alone did not affect animal performance in the MWM (Supplementary Table 4), but it completely blocked the THC-induced improvement of cognitive functions (Fig. 4a). It also abolished the THC-induced increase in AcH3K9, the increased expression of synapsin I (Fig. 4b), the increase in Bdnf and Kl mRNA expression (Fig. 4c), and the decrease in Casp1 or Ctgf expression (Supplementary Fig. 9). These data demonstrate that the beneficial effects of low-dose THC administration are dependent on
an epigenetic mechanism involving histone acetylation. This is in line with previous findings showing that enhanced histone acetylation can result in recovery of cognitive abilities in old mice37.
We finally asked to what extent the effects of THC are mediated by the CB1 receptor. Disrupted CB1 signaling in Cb1−/− (Cnr1−/−) mice initially leads to superior learning and memory performance at a young age, but then to a rapid decline in cognitive abilities. Cb1−/− mice aged 5 months already show striking impairments in a broad range of memory models38 accompanied by typical histological and molecular markers of old age, such as loss of hippocampal neurons, increased inflammation markers39 and disrupted proteostasis40. When THC or vehicle was infused from minipumps into
mature Cb1−/− mice, it had no effect on their behavior in the MWM and did not influence gene expression, synapsin I densities or histone acetylation (Supplementary Fig. 10), thus indicating that the beneficial effects of THC are dependent on CB1 signaling. Furthermore, we also treated mice lacking CB1 on forebrain glutamatergic principal neurons, Neurod6-Cre; Cb1−/− mice, which show no age-related phenotypes41. In these animals, THC administration also had no effect
on the acquisition or reversal phase in the MWM test (Fig. 4d), and it did not change synapsin I protein levels, AcH3K9 (Fig. 4e), or the mRNA levels of Bdnf and Kl (Fig. 4f), Casp1 or Ctgf (Supplementary Fig. 10f). Thus, the effects of THC on cognitive performance, synaptogenesis, histone acetylation and the expression of age-related genes were all dependent on the CB1 signaling of forebrain glutamatergic neurons. The results do not exclude the possibility that CB1 receptors on other neurons are also involved.
Attempts to reverse age-related epigenetic processes through a pharmacological blockade of histone deacetylases have shown some promise in rodents33,42, but the deleterious side-effects have prevented application in humans43. Consequently, the generalized inhibition of histone deacetylation is not further considered to be a suitable treatment of
age-related pathologies. In contrast, cannabis preparations and THC are used for medicinal purposes. They have an excellent safety record and do not produce adverse side-effects when administered at a low dose to older individuals. Thus, chronic, low-dose treatment with THC or cannabis extracts could be a potential strategy to slow down or even to reverse cognitive decline in the elderly.
METHoDS
Methods, including statements of data availability and any associated accession codes and references, are available in the online version of the paper.
Note: Any Supplementary Information and Source Data files are available in the
online version of the paper.
This work was supported by the Deutsche Forschungsgemeinschaft grants FOR926
(SP2 and CP2), BI-1227/5 and SFB645. J.L.S. and A.Z. are members of the DFG
Cluster of Excellence ImmunoSensation.
AUTHOR Contributions
O.A., A.B.-G., J.L.S., M.D.-G., I.B. and A.Z. designed research; O.A., A.P., S.I., T.U.,
H.O., I.R., K.M., A.D. and A.B.-G. performed research; O.A., A.P., A.B.-G., T.U.,
J.L.S. and A.Z. analyzed data; and O.A., A.B.-G., J.L.S. and A.Z. wrote the paper.
The authors declare no competing financial interests.
Reprints and permissions information is available online at http://www.nature.com/
reprints/index.html.
- Di Marzo, V., Stella, N. & Zimmer, A. Endocannabinoid signalling and the
deteriorating brain. Nat. Rev. Neurosci. 16, 30–42 (2015). - Bilkei-Gorzo, A. The endocannabinoid system in normal and pathological brain
ageing. Phil. Trans. R. Soc. Lond. B 367, 3326–3341 (2012). - Wang, L., Liu, J., Harvey-White, J., Zimmer, A. & Kunos, G. Endocannabinoid
signaling via cannabinoid receptor 1 is involved in ethanol preference and its age-
dependent decline in mice. Proc. Natl. Acad. Sci. USA 100, 1393–1398 (2003). - Berrendero, F. et al. Changes in cannabinoid receptor binding and mRNA levels in
several brain regions of aged rats. Biochim. Biophys. Acta 1407, 205–214
(1998). - Romero, J. et al. Loss of cannabinoid receptor binding and messenger RNA levels
and cannabinoid agonist–stimulated [35S]guanylyl-5′O-(thio)-triphosphate binding
in the basal ganglia of aged rats. Neuroscience 84, 1075–1083 (1998). - Piyanova, A. et al. Age-related changes in the endocannabinoid system in the mouse
hippocampus. Mech. Ageing Dev. 150, 55–64 (2015). - Han, J. et al. Acute cannabinoids impair working memory through astroglial CB1
receptor modulation of hippocampal LTD. Cell 148, 1039–1050 (2012). - Puighermanal, E., Busquets-Garcia, A., Maldonado, R. & Ozaita, A. Cellular and
intracellular mechanisms involved in the cognitive impairment of cannabinoids.
Phil. Trans. R. Soc. Lond. B 367, 3254–3263 (2012). - Varvel, S.A., Anum, E., Niyuhire, F., Wise, L.E. & Lichtman, A.H. ∆9-THC-induced
- cognitive deficits in mice are reversed by the GABAA antagonist bicuculline.
- Psychopharmacology (Berl.) 178, 317–327 (2005).
- Head, E. et al. Synaptic proteins, neuropathology and cognitive status in the oldest-
old. Neurobiol. Aging 30, 1125–1134 (2009). - Morrison, J.H. & Baxter, M.G. The ageing cortical synapse: hallmarks and
implications for cognitive decline. Nat. Rev. Neurosci. 13, 240–250 (2012). - Duce, J.A. et al. Gene profile analysis implicates Klotho as an important contributor
to aging changes in brain white matter of the rhesus monkey. Glia 56, 106–117
(2008). - Kurosu, H. et al. Suppression of aging in mice by the hormone Klotho. Science
309, 1829–1833 (2005). - Semba, R.D. et al. Plasma klotho and mortality risk in older community-dwelling
adults. J. Gerontol. A Biol. Sci. Med. Sci. 66, 794–800 (2011). - Dubal, D.B. et al. Life extension factor klotho enhances cognition. Cell Rep. 7,
1065–1076 (2014). - Cuenco, K.T. et al. Association of TTR polymorphisms with hippocampal atrophy
in Alzheimer disease families. Neurobiol. Aging 32, 249–256 (2011). - Li, X. & Buxbaum, J.N. Transthyretin and the brain re-visited: is neuronal synthesis
of transthyretin protective in Alzheimer’s disease? Mol. Neurodegener. 6, 79
(2011). - Tanaka, J. et al. Protein synthesis and neurotrophin-dependent structural plasticity
of single dendritic spines. Science 319, 1683–1687 (2008). - Neidl, R. et al. Late-life environmental enrichment induces acetylation events and
nuclear factor κB–dependent regulations in the hippocampus of aged rats showing
improved plasticity and learning. J. Neurosci. 36, 4351–4361 (2016). - Erickson, K.I. et al. Exercise training increases size of hippocampus and improves
memory. Proc. Natl. Acad. Sci. USA 108, 3017–3022 (2011). - Gemma, C. & Bickford, P.C. Interleukin-1β and caspase-1: players in the regulation
of age-related cognitive dysfunction. Rev. Neurosci. 18, 137–148 (2007). - Khodosevich, K. et al. Connective tissue growth factor regulates interneuron survival
and information processing in the olfactory bulb. Neuron 79, 1136–1151
(2013). - Robison, A.J. & Nestler, E.J. Transcriptional and epigenetic mechanisms of
addiction. Nat. Rev. Neurosci. 12, 623–637 (2011). - Derkinderen, P. et al. Regulation of extracellular signal–regulated kinase by
cannabinoids in hippocampus. J. Neurosci. 23, 2371–2382 (2003). - Valjent, E. et al. ∆9-tetrahydrocannabinol-induced MAPK/ERK and Elk-1 activation
in vivo depends on dopaminergic transmission. Eur. J. Neurosci. 14, 342–352
(2001). - Impey, S., Obrietan, K. & Storm, D.R. Making new connections: role of ERK/MAP
kinase signaling in neuronal plasticity. Neuron 23, 11–14 (1999). - Kandel, E.R. The molecular biology of memory: cAMP, PKA, CRE, CREB-1,
CREB-2, and CPEB. Mol. Brain 5, 14 (2012). - Villeda, S.A. et al. Young blood reverses age-related impairments in cognitive
function and synaptic plasticity in mice. Nat. Med. 20, 659–663 (2014). - Fusco, S. et al. A role for neuronal cAMP responsive-element binding (CREB)-1 in
brain responses to calorie restriction. Proc. Natl. Acad. Sci. USA 109, 621–626
(2012). - Saura, C.A. & Valero, J. The role of CREB signaling in Alzheimer’s disease and
other cognitive disorders. Rev. Neurosci. 22, 153–169 (2011). - West, A.E. et al. Calcium regulation of neuronal gene expression. Proc. Natl. Acad.
Sci. USA 98, 11024–11031 (2001). - Das, C. et al. Binding of the histone chaperone ASF1 to the CBP bromodomain promotes
histone acetylation. Proc. Natl. Acad. Sci. USA 111, E1072–E1081 (2014). - Vecsey, C.G. et al. Histone deacetylase inhibitors enhance memory and synaptic
plasticity via CREB:CBP-dependent transcriptional activation. J. Neurosci. 27,
6128–6140 (2007). - Shieh, P.B., Hu, S.C., Bobb, K., Timmusk, T. & Ghosh, A. Identification of a
signaling pathway involved in calcium regulation of BDNF expression. Neuron 20,
727–740 (1998). - Gao, H. et al. Long-term dietary α-linolenic acid supplement alleviates cognitive
impairment correlate with activating hippocampal CREB signaling in natural aging
rats. Mol. Neurobiol. 53, 4772–4786 (2016) - Gräff, J. & Tsai, L.H. Histone acetylation: molecular mnemonics on the chromatin.
Nat. Rev. Neurosci. 14, 97–111 (2013). - Peleg, S. et al. Altered histone acetylation is associated with age-dependent memory
impairment in mice. Science 328, 753–756 (2010). - Bilkei-Gorzo, A. et al. Early age-related cognitive impairment in mice lacking
cannabinoid CB1 receptors. Proc. Natl. Acad. Sci. USA 102, 15670–15675
(2005). - Albayram, O. et al. Role of CB1 cannabinoid receptors on GABAergic neurons in
brain aging. Proc. Natl. Acad. Sci. USA 108, 11256–11261 (2011). - Piyanova, A. et al. Loss of CB1 receptors leads to decreased cathepsin D levels
and accelerated lipofuscin accumulation in the hippocampus. Mech. Ageing Dev.
134, 391–399 (2013). - Monory, K. et al. The endocannabinoid system controls key epileptogenic circuits
in the hippocampus. Neuron 51, 455–466 (2006). - Fischer, A., Sananbenesi, F., Wang, X., Dobbin, M. & Tsai, L.H. Recovery of learning
and memory is associated with chromatin remodelling. Nature 447, 178–182
(2007). - Day, J.J. & Sweatt, J.D. Epigenetic treatments for cognitive impairments.
Neuropsychopharmacology 37, 247–260 (2012).
This originally appeared at https://www.researchgate.net/publication/316842775_A_chronic_low_dose_of_D9-tetrahydrocannabinol_THC_restores_cognitive_function_in_old_mice