This originally appeared at https://pubmed.ncbi.nlm.nih.gov/22776349/
a b s t r a c t
Breast cancer is a very common disease that affects approximately 1 in 10 women at some point in their
lives. Importantly, breast cancer cannot be considered a single disease as it is characterized by distinct
pathological and molecular subtypes that are treated with different therapies and have diverse clinical
outcomes. Although some highly successful treatments have been developed, certain breast tumors are
resistant to conventional therapies and a considerable number of them relapse. Therefore, new strategies
are urgently needed, and the challenge for the future will most likely be the development of individual-
ized therapies that specifically target each patient’s tumor. Experimental evidence accumulated during
the last decade supports that cannabinoids, the active components of Cannabis sativa and their deriva-
tives, possess anticancer activity. Thus, these compounds exert anti-proliferative, pro-apoptotic, anti-
migratory and anti-invasive actions in a wide spectrum of cancer cells in culture. Moreover, tumor
growth, angiogenesis and metastasis are hampered by cannabinoids in xenograft-based and geneti-
cally-engineered mouse models of cancer. This review summarizes our current knowledge on the anti-
tumor potential of cannabinoids in breast cancer, which suggests that cannabinoid-based medicines
may be useful for the treatment of most breast tumor subtypes
Introduction
Cannabinoids are a family of unique compounds synthesized by
Cannabis sativa (marijuana) that have not been found as yet in any
other plant. The main representative cannabinoid, owing to its high
abundance in the plant and its strong biological activity, is D9
-tet-rahydrocannabinol (THC).1 For decades, and mainly due to their
lipophilic nature, it was assumed that cannabinoids exerted their
effects by perturbing the biophysical properties of biological mem-
branes.2 This scenario changed dramatically in 1990, when the first
cannabinoid-specific membrane receptor (CB 1) was characterized
and cloned.3 This discovery boosted the scientific research on
cannabinoids, which currently constitutes a very active field in
biomedicine. The term cannabinoid includes now not only the
marijuana-derived compounds that are structurally related to
THC (phytocannabinoids), but also the synthetic molecules that
activate the same primary targets as THC (synthetic cannabinoids)
and a family of endogenously produced compounds that engage
cannabinoid receptors (endocannabinoids), of which anandamide
(arachidonoylethanolamide, AEA)4 and 2-arachidonoylglicerol
(2-AG)5,6 are the main – if not only – representatives. Two G protein-coupled receptors (GPCRs) that are selectively engaged
by cannabinoids have been cloned so far (CB1 and CB2), 3,7 and some
evidence indicates that cannabinoids may act by binding to addi-
tional receptors such as the vanilloid receptor 1 (TRPV1) and the
orphan GPCR GPR55.8 The endocannabinoids, together with their
receptors and the proteins in charge of their transport, synthesis
and degradation, constitute the so called endocannabinoid system,
which is involved in the control of a wide variety of biological func-
tions such as motor behavior, memory and learning, pain, the
immune response or bone physiology, just to mention a few.2
Cannabinoids and cancer
The therapeutic properties of marijuana have been known for
millennia, but the use in the clinic of either plant-derived prepara-
tions or pure cannabinoids is still very limited. To date, only three
cannabinoid-based medicines can be prescribed, and for very spe-
cific indications. The orexigenic and anti-cachexic properties of can-
nabinoids are exploited by MarinolÒ (oral capsules of dronabinol –
synthetically generated THC) to manage weight loss associated to
wasting syndrome in patients with AIDS. SativexÒ (nabiximols, an
oromucosal spray containing plant extracts enriched in THC and
cannabidiol at an approximate 1:1 ratio) has been recently approved
in several European countries, Canada and New Zealand for the re-
lief of spasticity associated to multiple sclerosis, and in Canada for
the treatment of neuropathic pain in the same disease. In the
context of cancer, it is well established that cannabinoids have
antiemetic properties,9 and, in fact, MarinolÒ and CesametÒ (oral
capsules of nabilone – a synthetic THC analog) can be prescribed
to prevent nausea and vomiting elicited by standard chemothera-
peutic regimes. In addition, SativexÒ is approved in Canada for the
treatment of cancer-associated pain.
Aside from these palliative actions, recent preclinical evidence
suggests that cancer patients might benefit from cannabinoids in
an additional manner: since the late 1990s, an important amount
of experimental data has shown that these compounds exert
anti-tumor effects in different models of cancer, ranging from cell
cultures to xenografted and genetically engineered mice.10
Cannabinoid anti-tumor action relies on the blockade of several
hallmarks of tumor progression. Thus, they inhibit uncontrolled
cancer cell growth (by inhibiting cancer cell proliferation and by
inducing cancer cell death by apoptosis) and impair tumor angio-
genesis and metastasis. Interestingly, some – if not all – of these
effects have been observed in tumor cells from very different
origin, including gliomas, melanomas, carcinomas of the breast,
skin, lungs, liver, pancreas, colon, prostate, and lymphomas
amongst others, which indicates that cannabinoid anti-tumor
action has a general rather than tumor-type specific nature.10
Anti-tumor effects of cannabinoids in breast cancer
Breast cancer is the most common malignant disease among
Western women. Although the rates of mortality have declined
since the late 1990s, mainly due to adjuvant systemic therapy
and earlier detection by palpation and mammograms, certain
breast tumors remain resistant to conventional therapies. In addi-
tion, current treatments have side effects that substantially affect
the patients’ quality of life. 11–13 It is therefore obvious that new
therapeutic strategies are needed for the management of this con-
dition. As breast cancer is an extremely heterogeneous disease that
comprises tumors that are very diverse in terms of molecular por-
traits, prognosis and treatments,13 in this review we will distin-
guish the three main breast cancer subtypes according to
classical molecular profiles: hormone receptor-positive, HER2-po-
sitive and triple-negative tumors. Although the strength of the
experimental data is different in each case, evidence obtained so
far suggests that cannabinoid-based medicines may be useful for
the treatment of these three breast cancer subtypes.
Cannabinoids and hormone-sensitive breast cancer
The presence of estrogen receptors (ER) and/or progesterone
receptors (PR) in breast cancer cells, as determined by immunohis-
tochemistry-based methods, defines a subgroup of breast tumors
that may respond to endocrine therapy. Specifically, these patients
are treated with surgical and/or pharmacological approaches that
block estrogenic signaling, which has pro-proliferative and pro-
survival features. The targeted strategies include the removal of
the endogenous source of estrogens (the ovaries) and/or the use
of selective estrogen receptor modulators, such as the widely used
tamoxifen, or inhibitors of aromatase, the main enzyme responsi-
ble for estrogen synthesis.14
It has been demonstrated that cannabinoids modulate pivotal
tumor progression-related aspects of ER+
/PR + breast cancer cells.
Thus, anandamide inhibits basal 15,16 and prolactin- and nerve
growth factor (NGF)-induced proliferation15,17 of MCF-7 and
EFM-19 cells in culture. This effect is mediated by the activation
of CB1 receptors 15–17 and is not accompanied by cancer cell
death.15 Anandamide produces this anti-proliferative action by
blocking the progression through the cell cycle, specifically by pre-
venting the transition from the G1 to the S phase,15 and by inhib-
iting adenylyl cyclase and thus activating the Raf-1/ERK/MAPK
cascade, which, upon sustained activation, ultimately down-regu-
lates prolactin and TrkA NGF receptors15–17 (Fig. 1).
The proliferation of the ER/PR+ human breast cancer cell line
EVSA-T is also decreased in response to THC.18,19 Once again, the
cell cycle is targeted: a blockade in the transition from G2 to mito-
sis via CB2 receptors, produced by the inhibition of CDK1, was ob-
served.19 Cell cycle arrest is ensued by apoptotic cell death,18,19 and
the activation of the transcription factor JunD, owing to upregula-
tion of gene expression and translocation to the nucleus, is essen-
tial for these actions 18 (Fig. 1).
Besides cancer cell proliferation, cannabinoids impair ER +
/PR +
cancer cell migration and invasion in culture. Specifically, selective
activation of CB2 receptors in MCF-7 cells that overexpress the che-
mokine receptor CXCR4 inhibited chemotaxis and wound healing
as induced by the CXCR4 ligand CXCL1220 (Fig. 1). The CXCL12/
CXCR4 signaling axis plays a pivotal role in directing breast cancer
cells to distant sites21 and, therefore, the aforementioned finding
suggests that cannabinoids may modulate hormone-sensitive
breast cancer metastasis. However, the experimental support for
this notion is still weak and further research with more complex
models should be performed to validate it.
Cannabinoids and HER2-positive breast cancer
The breast tumors that express the tyrosine kinase receptor
HER2, as determined by immunohistochemistry and fluorescence
in situ hybridization (FISH) approaches, constitute another well de-
fined breast cancer histopathological subtype. HER2 belongs to the
epidermal growth factor receptor (EGFR) family, which consists of
four members (ErbB1/HER1/EGFR, ErbB2/HER2, ErbB3/HER3 and
ErbB4/HER4). They all have intrinsic tyrosine kinase activity and,
upon ligand binding and subsequent dimerization, they activate a
number of oncogenic processes, including cell proliferation and
survival.22 The outcome of these patients has considerably im-
proved since the incorporation to the clinics of HerceptinÒ (trast-
uzumab, a humanized monoclonal antibody against the
extracellular domain of HER2). Other compounds are in use or un-
der development to overcome trastuzumab resistance, the most
prominent being TykerbÒ (lapatinib), a dual EGFR/HER2 tyrosine
kinase inhibitor.13
Strong preclinical evidence suggests that cannabinoids may be
useful for the treatment of this particular subset of patients. Thus,
THC produces a significant anti-tumor action in MMTV-neu mice,23
a well established and clinically relevant model of HER2-positive
metastatic breast cancer.24 THC treatment reduces not only tumor
growth but also the number of tumors generated per animal and
the percentage of animals with lung metastases. 23 THC action re-
lies on the impairment of cancer cell proliferation, via inhibition
of the pro-tumorigenic kinase AKT, and on the induction of cancer
cell death by apoptosis. 23 A reduction in the number of tumor
blood vessels is also observed, suggesting that tumor angiogenesis
is impaired by THC23 (Fig. 2).
Xenograft-based approaches strengthen the hypothesis that
HER2-overexpressing tumors may be sensitive to cannabinoids.
Two different cell lines isolated from MMTV-neu-derived tumors
were injected either subcutaneously in immune-deficient mice23
or orthotopically in immune-competent syngenic FVB mice20 and
treated with THC23 and/or CB2-selective agonists.20,23 In both cases,
a significant reduction in tumor growth was observed, mediated by
the inhibition of AKT in one case23 and accompanied by a decrease
in the levels of activated ERK and CXCR4 in the other20 (Fig. 2).
Of interest, the anti-tumor effect of cannabinoids in all the
HER2-positive breast cancer models used so far is mediated by
the activation of CB2 receptors (Fig. 2). Thus, the anti-tumor action
of THC in the MMTV-neu model is mimicked by the CB2 -selective
agonist JWH-133.23 In the same line, the growth-inhibiting effect
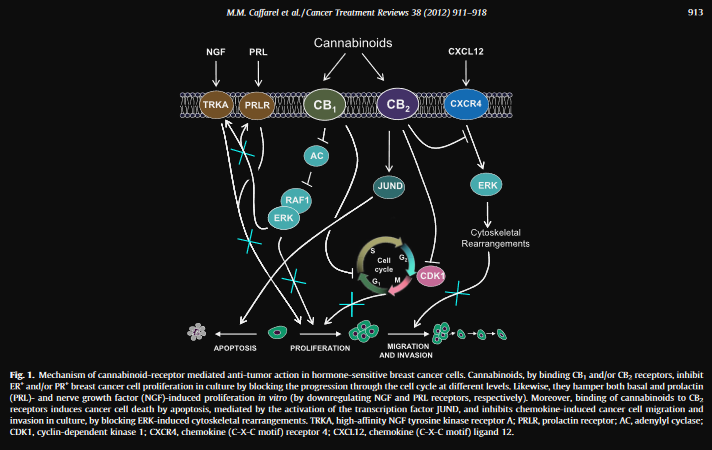
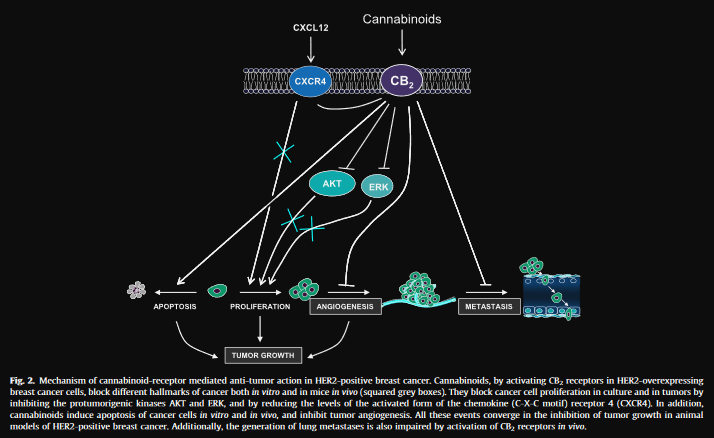
on orthotopic xenografts is produced by another CB2-selective ago-
nist (JWH-015)20 and the effect on subcutaneous xenografts is mimicked by JWH-133 and prevented by the CB2 -selective antago-
nist SR144528.23 The involvement of this particular receptor in
cannabinoid anti-tumor action may have important clinical impli-
cations since the psychotropic effects associated to these com-
pounds are mediated by the activation of CB1 (and not CB2 )
receptors present in the brain.25 Therefore, a CB2 -directed therapy
may be, in principle, efficacious in curbing the growth of these tu-
mors and would be devoid of the classical cannabis-associated psy-
chotropic side effects.
Cannabinoids and triple-negative breast cancer
The remaining group of breast tumors according to immunopath-
ological criteria is the triple-negative one. This definition refers to
the lack of expression of ER, PR and HER2. To date, there is no stan-
dard targeted therapy for these patients, whose prognosis is very
poor as a group.12 Efforts are being made to improve chemotherapy
responses and they include, for example, the combined use of
angiogenesis inhibitors such as AvastinÒ (bevacizumab) and poly
(adenosine diphosphate–ribose) polymerase (PARP) inhibitors.12,13
Both in vitro and in vivo preclinical evidence indicates that tri-
ple-negative breast cancer may be treated with cannabinoids. A
battery of synthetic cannabinoids has been tested in the human tri-
ple-negative breast cancer cell line MDA-MB-231, and all of them
produced an inhibition of cell proliferation.19,26–31 For example,
the metabolically stable analog of anandamide Met-F-AEA reduces
MDA-MB-231 proliferation without inducing cell death 32 by
arresting cells in the S phase of the cell cycle.26 This effect is
accompanied by a decrease in the activity of the cyclin-dependent
kinase CDK2 and the modulation of the levels of other important
cell cycle regulators.26 CB1 receptors seem to be the primary target
of Met-F-AEA action in this model26,32 (Fig. 3). The synthetic can-
nabinoids WIN 55,212-2 (a CB1 and CB2 mixed agonist) and JWH-
133 also produce an inhibition of MDA-MB-231 proliferation by
blocking the progression trough the cell cycle, but in this case (i)
the cells are retained in the G0/G1 compartment, (ii) this effect is
connected to apoptosis, and (iii) it is mediated by both CB1 and
CB2 and receptors30 (Fig. 3). Whether these differences are ago-
nist-specific or due to other experimental issues has not been
clarified.
Interestingly, the cannabinoid proliferation-inhibiting effect is
reproduced in vivo, both in a xenograft-based and in the PyMT
genetically engineered model of triple-negative breast cancer. 30
In these two models, a significant reduction in tumor growth is ob-
served upon JWH-133 treatment, and the analysis of the tumors
revealed a significant decrease in the number of cancer cells under-
going proliferation.30 Of note, decreased immunoreactivity of the
vascular marker CD31 was found in cannabinoid-treated tumors,
which suggests that tumor angiogenesis is also hampered by
JWH-133.30 In line with the idea that cannabinoids impact not only
proliferation but also other tumor progression-related features of
triple-negative cells, it was found that migration of MDA-MB-231
cells in culture is blocked by Met-F-AEA, WIN 55,212-2, JWH-133
and JWH-015.20,30,32,33 As for the effects on proliferation, Met-
AEA action on migration is mediated by CB 1 receptors,32,33 while
that of WIN 55,212-2 and the JWH compounds is mainly produced
by activation of CB2 receptors20,30 (Fig. 3). Engagement of CB1
receptors by Met-F-AEA leads to the inhibition of the focal adhe-
sion kinase (FAK)/Src32 and RhoA-ROCK pathways, 33 while the
inhibitory effect produced by WIN 55,212-2 or JWH-133 via CB 2
receptors is accompanied by the inhibition of the COX-2/PGE2
axis 30 (Fig. 3). Both signaling systems play important roles in driv-
ing cell migration and metastasis.34–36 Finally, JWH-015 reduces
CXCL12-induced cell migration and invasion of a highly metastatic
MDA-MB-231-derived cell line by inhibiting ERK and cytoskeletal
focal adhesion and stress fiber formation via CB2 receptors20
(Fig. 3), the latter being crucial events in cancer invasion and
metastasis.37
Together, these observations suggest that cannabinoids, via CB1
and/or CB2 receptors, confer a less invasive phenotype to triple-
negative breast cancer cells in culture, and allows hypothesizing
that these compounds may reduce the cancer cell metastatic po-
tential in vivo. Importantly, this hypothesis has been confirmed
in an animal model of lung metastasis. Thus, WIN 55,212-2 and
JWH-133 reduces the number of lung metastases generated by
injection of MDA-MB-231 cells into the lateral tail vein, an effect
that is completely prevented by the combined pharmacological
blockade of CB1 and CB2 receptors30 (Fig. 3).
Phytocannabinoids other than THC have been shown as well to
exert anti-tumor actions in breast cancer. The most studied in the
triple-negative context has been cannabidiol (CBD). This com-
pound displays low affinity for CB1 and CB2 receptors8 and,
although its mechanism of action is not completely understood,
it is emerging as an attractive potential therapeutic tool for a num-
ber of conditions.38,39 Several groups have reported that CBD re-
duces MDA-MB-231 cell proliferation in culture, but conflicting
results were obtained as regards the molecular bases underlying
this effect, especially concerning cannabinoid receptor involve-
ment. Ligresti and coworkers observed an induction of apoptosis
that was partially prevented by CB2 and TRPV1 antagonists.27
Based on these results and previous data from the same group,
the authors proposed that CBD action is produced by the combina-
tion of the direct activation of TRPV1 receptors by CBD, the indirect
activation of CB2 receptors by anandamide (whose deactivation is
inhibited by CBD)40 and the activation of other uncharacterized
and unique targets of CBD.27 On the other hand, a recent paper
rules out the involvement of CB1, CB2 and TRPV1 receptors in
CBD-induced apoptosis of MDA-MB-231 cells.31 In this case, CBD
induces endoplasmic reticulum (ER) stress and a subsequent inhi-
bition of the AKT/mTORC1 axis that leads to autophagy and mito-
chondria-driven apoptosis.31 The induction of ER stress and/or
autophagy followed by apoptosis has been previously associated
to cannabinoid anti-tumor action in different types of cells, includ-
ing glioma,41 pancreatic cancer,41,42 hepatocellular carcinoma,43
rhabdomyosarcoma44 and mantle cell lymphoma,45 which sug-
gests that this may be a general mechanism of cancer cell death in-
duced by cannabinoids. 10
Within the complex and controversial mechanistic explanations
for CBD action in cancer cells, the increase in reactive oxygen spe-
cies (ROS) seems to be the most accepted and reproducible.
Although it is known that CBD per se is a potent antioxidant,38 it
has been observed that it triggers a signaling mechanism that in-
volves the generation of ROS in glioma,46 leukemia47 and MDA-
MB-231 breast cancer cells. 27–29,31 The molecular explanation for
this apparent divergence has not been elucidated yet.
The inhibition of breast cancer cell proliferation by CBD has
been corroborated in vivo. Treatment of subcutaneous xenografts
generated from MDA-MB-231 cells in immune-deficient mice re-
sulted in a marked reduction of tumor growth.27 Similar results
were observed in orthotopic xenografts generated from 4T1 tri-
ple-negative breast cancer murine cells in syngenic BALB/c mice,
although in this case tumors acquired resistance to CBD after
3 weeks of treatment and, from that time onwards, grew as fast
as the vehicle-treated tumors at the end of the experiment.29
As for other cannabinoids, CBD action on triple-negative breast
cancer cells impacts not only proliferation but also metastasis-re-
lated capabilities. Thus, MDA-MB-231 and 4T1 cell invasion was
hampered in culture by CBD.28,29 Most important, this compound
reduced the number of lung metastases generated by intraplantar
injection of MDA-MB-231 cells, 31 by injection of 4T1 cells into the
tail vein, and by spontaneous formation from 4T1 orthotopic
xenografts. 29 The down-regulation of Id1 (an inhibitor of basic he-
lix-loop-helix transcription factors) played a pivotal mechanistic
role in these CBD anti-tumor actions.28,29
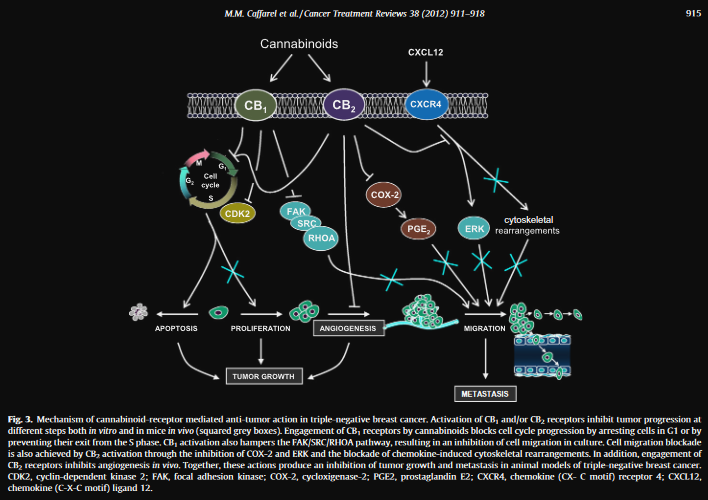
Pro-tumor effects of cannabinoids in breast cancer
Although the vast majority of reports published so far show that
cannabinoids induce anti-tumor responses not only in breast but in
many other types of cancer, a reduced number of articles have
reported pro-tumor actions in response to cannabinoids. Thus,
McKallip and coworkers found that THC, via CB2 receptors,
enhanced the growth of 4T1-derived xenografts and metastases
by the inhibition of the anti-tumor immune response.48 Similarly,
the growth of xenografted lung tumors was augmented by THC
via CB2 receptors49 and by methanandamide via a receptor-
independent mechanism.50 However, other reports demonstrate
an important contribution of the immune system in cannabinoid-
induced anti-tumor action. For example, the growth of melanoma
xenografts was inhibited more efficiently by cannabinoids in
immune-competent mice than in immune-deficient mice.51 In addi-
tion, a prolonged treatment of immune-competent rats with THC
decreased the incidence of tumors and enhanced overall animal sur-
vival.52 Additional studies should be performed to clarify whether
cannabinoids activate or inhibit the immune surveillance of tumors.
Takeda and coworkers reported that THC enhances the prolifer-
ation of MCF-7 cells in culture.53,54 Interestingly, both in this case
as in the work by McKallip and coworkers, 48 the cells in which
pro-tumor effects of cannabinoids were observed expressed unde-
tectable/very low levels of cannabinoid receptors. It would be
important to confirm this observation in other breast cancer cell
lines to determine whether the lack of CB1/CB 2 receptors could
be a marker of resistance to cannabinoid anti-tumor action.
Additionally, the pro-tumor effect of cannabinoids in certain
conditions could be explained by the biphasic effects that these
compounds have in multiple processes such as the control of appetite or anxiety.55 Indeed, we have observed a biphasic effect of cannabinoids in the proliferation of various cancer cell lines: while low
doses of cannabinoids promote cell proliferation (unpublished
observations), higher doses exert the well described anti-prolifera-
tive effect. However, only two works have reported so far pro-tu-
mor effects of cannabinoids in vivo. 48,50
Expression of cannabinoid receptors in human breast tumors
There is compelling evidence demonstrating the expression of
cannabinoid receptors in human breast cancer biopsies. 19,23,30 Of
interest, a correlation between CB 2 receptor expression and tumor
aggressiveness has been found. 19 Thus, the levels of CB2 mRNA
were higher in ER/PR tumors than in ER +
/PR tumors, in HER2-
positive tumors than in HER2-negative tumors, and in high histo-
logical grade than in low histological grade tumors. 19 This associa-
tion between cannabinoid receptor expression and tumor
aggressiveness has been observed as well in other types of tumors
such as gliomas, 56–58 prostate59 and colorectal cancers, 60 which
may indicate that the endocannabinoid system is up-regulated in
cancer. It is not clear yet whether this up-regulation is protective
or pro-tumorigenic, and this question certainly deserves further
investigation. To address this issue, it would be interesting to ana-
lyze, for example, the levels of endocannabinoids as well as of their
metabolizing enzymes in breast tumors, and whether the lack of
cannabinoid receptors in mice reduces or enhances breast tumor
generation and/or progression. Conflicting reports have been pub-
lished in other types of cancer, showing for example that the lack
of cannabinoid receptors in mice protects from skin carcinogene-
sis61 but accelerates intestinal cancer growth.62
Of interest, the sensitivity of human breast cancer cells to cannabinoids in culture correlates with their aggressiveness. For example, we showed that ER- cell lines were more susceptible to
cannabinoid treatment than ER+ cells.19 In addition, Grimaldi
et al. observed that Met-F-AEA inhibited the proliferation of the
highly metastatic MDA-MB-231 cell line more efficiently than that
of the poorly invasive and non-metastatic T47D cell line.32
Other potential benefits of cannabinoids for the treatment of
breast cancer
A general feature of cannabinoid anti-tumor action in breast
and other types of tumors is the lack of toxicity on non-tumor cells.
Thus, the inhibition of cancer cell proliferation upon cannabinoid
treatment was not evident in non-transformed human mammary
epithelial cells.18,19,27,31 This observation, that has been made as
well in other types of cells such glioma cells/astrocytes,41,63,64 skin
carcinoma cells/keratinocytes65 and melanoma cells/melano-
cytes,51 has not been mechanistically explained yet, but can be
mostly attributed to different cannabinoid receptor-triggered
intracellular signaling events in cancer versus non-cancer cells
rather than to different expression patterns of cannabinoid recep-
tors between both kinds of cells.10,19,64
An additional characteristic of cannabinoids, which may have
important clinical implications, is their safety. Cannabinoid-based
medicines have been proven very safe in thousands of patients en-
rolled in multiple clinical trials along the last years and in the can-
cer patients that use them for the management of pain, nausea and
vomiting.66–68 Moreover, the safety of THC on recurrent glioblas-
toma multiforme patients was confirmed in a pilot clinical trial.69
In all these cases, the most reported side effects were mild/moder-
ate dizziness and fatigue.70
The most realistic approach to introduce new therapeutic
agents in clinical oncology is their combination with standard
treatments. There is preclinical evidence showing that the combi-
nation of cannabinoids with other established anticancer agents
not only does not have negative effects but, instead, induces a syn-
ergistic action. For example, temozolomide (the standard therapy
for glioma patients) exerted an anti-tumor effect in animal models
that was profoundly improved by combination with cannabi-
noids.71 Of interest, temozolomide/cannabinoid combinations
were very effective in reducing the growth of temozolomide-resis-
tant glioma cell lines.71 Combination of cannabinoids with other
anticancer agents such as gemcitabine, paclitaxel and 5-fluoroura-
cil had synergistic inhibitory effects on the proliferation of cultured
pancreatic,72 gastric73 and colorectal74 cancer cells, respectively.
As mentioned above, CBD induces marked anti-tumor actions in
models of triple-negative breast cancer and other cancers. The
addition of this particular compound to potential cannabinoid-
based anticancer therapies would have additional benefits. First,
it has been reported that CBD enhances the activity of THC in
inhibiting the growth of glioma cells in culture71,75 and in xeno-
grafts,71 so it is tempting to speculate that this could also be the
case for breast cancer. Second, as mentioned above, CBD does not
bind with significant affinity to CB1 receptors and, therefore, it does
not exert psychoactivity25 – moreover, it may attenuate some of
the psychoactive effects of THC.38 Third, CBD exerts by itself a
plethora of therapeutic effects [including anxiolytic, antipsychotic,
antiepileptic, analgesic, anti-inflamatory, anti-ischemic, neuropro-
tective and antiemetic effects38 ] in animal models, some of which
might be positive for cancer patients.
Conclusions
There is compelling evidence showing that cannabinoids have
anti-tumor activity in preclinical models of breast cancer. These
data come not only from cell culture systems but also from more
complex and clinically relevant animal models. This anti-tumor ac-
tion is produced by the blockade of several hallmarks of cancer
(sustained cancer cell proliferation, metastasis and angiogenesis)
rather than by the targeting of a unique process, and the com-
pounds are not only effective but safe. In order to take the next
steps towards potential clinical trials our knowledge on several
issues should be improved. For example, although the three histo-
pathological subtypes of breast cancer seem to respond to cannab-
inoids, it would be important to define which the most appropriate
patient population for cannabinoid-based therapies is. To date, the
most solid preclinical evidence (because of the robustness of the
models used and the mechanistic information obtained) points to
HER2-positive and triple-negative tumors. Another important
question is which cannabinoid/s is/are the best to be tested in pa-
tients. In our opinion, the most reasonable candidate would be
adding to a standard chemotherapy or immunotherapy a mixture
of a cannabinoid targeting CB1 and/or CB2 receptors plus CBD. This
combination would have the advantage of two cannabinoid com-
pounds acting through different mechanisms of action that would
theoretically produce (as it has been demonstrated in mice bearing
gliomas) a cooperating anti-tumor effect. In addition, the presence
of CBD in the drug cocktail would possibly attenuate non-desired
effects of psychoactive cannabinoids and would provide some of
the palliative effects that this compound exerts per se.
Conflict of interest
Dr. Cristina Sánchez and Dr. Manuel Guzmán disclose that they
have received research support funding from GW Pharmaceuticals.
The rest of the authors disclose no conflict of interest.
Acknowledgements
This work was supported by Grants from Ministerio de
Economía y Competitividad (PI11/00295 to C.S.), Comunidad de
Madrid (S2011/BMD-2308 and 950344 to M.G.) and GW Pharma-
ceuticals (to C.S.). C.A. and E.P.-G. are recipients of a predoctoral
fellowship from Ministerio de Economía y Competitividad and of
a postdoctoral research contract from Fundación Científica Aso-
ciación Española Contra el Cáncer, respectively.
References
1. Gaoni Y, Mechoulam R. Isolation, structure and partial synthesis of an active
constituent of hashish. J Am Chem Soc 1964;86:1646–7.
2. Pacher P, Batkai S, Kunos G. The endocannabinoid system as an emerging target
of pharmacotherapy. Pharmacol Rev 2006;58:389–462.
3. Matsuda LA, Lolait SJ, Brownstein MJ, et al. Structure of a cannabinoid receptor
and functional expression of the cloned cDNA. Nature 1990;346:561–4.
4. Devane WA, Hanus L, Breuer A, et al. Isolation and structure of a brain
constituent that binds to the cannabinoid receptor. Science 1992;258:1946–9.
5. Mechoulam R, Ben-Shabat S, Hanus L, et al. Identification of an endogenous 2-
monoglyceride, present in canine gut, that binds to cannabinoid receptors.
Biochem Pharmacol 1995;50:83–90.
6. Sugiura T, Kondo S, Sukagawa A, et al. 2-Arachidonoylglycerol: a possible
endogenous cannabinoid receptor ligand in brain. Biochem Biophys Res Commun
1995;215:89–97.
7. Munro S, Thomas KL, Abu-Shaar M. Molecular characterization of a peripheral
receptor for cannabinoids. Nature 1993;365:61–5.
8. Pertwee RG, Howlett AC, Abood ME, et al. International Union of Basic and
Clinical Pharmacology. LXXIX. Cannabinoid receptors and their ligands: beyond
CB and CB. Pharmacol Rev 2010;62:588–631.
9. Machado Rocha FC, Stefano SC, De Cassia Haiek R, et al. Therapeutic use of
Cannabis sativa on chemotherapy-induced nausea and vomiting among cancer
patients: systematic review and meta-analysis. Eur J Cancer Care (Engl)
2008;17:431–43.
10. Velasco G, Sánchez C, Guzmán M. Towards the use of cannabinoids as
antitumour agents. Nat Rev Cancer 2012.
11. DeSantis C, Siegel R, Bandi P, et al. Breast cancer statistics, 2011. CA Cancer J Clin
2011;61:409–18.
12. Foulkes WD, Smith IE, Reis-Filho JS. Triple-negative breast cancer. N Engl J Med
2010;363:1938–48.
13. Higgins MJ, Baselga J. Targeted therapies for breast cancer. J Clin Invest
2011;121:3797–803.
14. Nilsson S, Koehler KF, Gustafsson JA. Development of subtype-selective
oestrogen receptor-based therapeutics. Nat Rev Drug Discov 2011;10:
778–92.
15. De Petrocellis L, Melck D, Palmisano A, et al. The endogenous cannabinoid
anandamide inhibits human breast cancer cell proliferation. Proc Natl Acad Sci U
S A 1998;95:8375–80.
16. Melck D, Rueda D, Galve-Roperh I, et al. Involvement of the cAMP/protein
kinase A pathway and of mitogen-activated protein kinase in the anti-
proliferative effects of anandamide in human breast cancer cells. FEBS Lett
1999;463:235–40.
17. Melck D, De Petrocellis L, Orlando P, et al. Suppression of nerve growth factor
Trk receptors and prolactin receptors by endocannabinoids leads to inhibition
of human breast and prostate cancer cell proliferation. Endocrinology
2000;141:118–26.
18. Caffarel MM, Moreno-Bueno G, Cerutti C, et al. JunD is involved in the
antiproliferative effect of Delta(9)-tetrahydrocannabinol on human breast
cancer cells. Oncogene 2008;27:5033–44.
19. Caffarel MM, Sarrio D, Palacios J, et al. Delta9-tetrahydrocannabinol inhibits
cell cycle progression in human breast cancer cells through Cdc2 regulation.
Cancer Res 2006;66:6615–21.
20. Nasser MW, Qamri Z, Deol YS, et al. Crosstalk between chemokine receptor
CXCR4 and cannabinoid receptor CB2 in modulating breast cancer growth and
invasion. PLoS One 2011;6:e23901.
21. Zlotnik A, Burkhardt AM, Homey B. Homeostatic chemokine receptors and
organ-specific metastasis. Nat Rev Immunol 2011;11:597–606.
22. Zhang H, Berezov A, Wang Q, et al. ErbB receptors: from oncogenes to targeted
cancer therapies. J Clin Invest 2007;117:2051–8.
23. Caffarel MM, Andradas C, Mira E, et al. Cannabinoids reduce ErbB2-driven
breast cancer progression through Akt inhibition. Mol Cancer 2010;9:196.
24. Ursini-Siegel J, Schade B, Cardiff RD, et al. Insights from transgenic mouse
models of ERBB2-induced breast cancer. Nat Rev Cancer 2007;7:389–97.
25. Pertwee RG. Emerging strategies for exploiting cannabinoid receptor agonists
as medicines. Br J Pharmacol 2009;156:397–411.
26. Laezza C, Pisanti S, Crescenzi E, et al. Anandamide inhibits Cdk2 and activates
Chk1 leading to cell cycle arrest in human breast cancer cells. FEBS Lett
2006;580:6076–82.
27. Ligresti A, Moriello AS, Starowicz K, et al. Antitumor activity of plant
cannabinoids with emphasis on the effect of cannabidiol on human breast
carcinoma. J Pharmacol Exp Ther 2006;318:1375–87.
28. McAllister SD, Christian RT, Horowitz MP, et al. Cannabidiol as a novel inhibitor
of Id-1 gene expression in aggressive breast cancer cells. Mol Cancer Ther
2007;6:2921–7.
29. McAllister SD, Murase R, Christian RT, et al. Pathways mediating the effects of
cannabidiol on the reduction of breast cancer cell proliferation, invasion, and
metastasis. Breast Cancer Res Treat 2011.
30. Qamri Z, Preet A, Nasser MW, et al. Synthetic cannabinoid receptor agonists
inhibit tumor growth and metastasis of breast cancer. Mol Cancer Ther
2009;8:3117–29.
31. Shrivastava A, Kuzontkoski PM, Groopman JE, et al. Cannabidiol induces
programmed cell death in breast cancer cells by coordinating the cross-talk
between apoptosis and autophagy. Mol Cancer Ther 2011;10:1161–72.
32. Grimaldi C, Pisanti S, Laezza C, et al. Anandamide inhibits adhesion and
migration of breast cancer cells. Exp Cell Res 2006;312:363–73.
33. Laezza C, Pisanti S, Malfitano AM, et al. The anandamide analog, Met-F-AEA,
controls human breast cancer cell migration via the RHOA/RHO kinase
signaling pathway. Endocr Relat Cancer 2008;15:965–74.
34. Hoellen F, Kelling K, Dittmer C, et al. Impact of cyclooxygenase-2 in breast
cancer. Anticancer Res 2011;31:4359–67.
35. Jaffe AB, Hall A. Rho GTPases: biochemistry and biology. Annu Rev Cell Dev Biol
2005;21:247–69.
36. Narumiya S, Tanji M, Ishizaki T. Rho signaling, ROCK and mDia1, in
transformation, metastasis and invasion. Cancer Metastasis Rev 2009;28:
65–76.
37. Hall A. The cytoskeleton and cancer. Cancer Metastasis Rev 2009;28:5–14.
38. Izzo AA, Borrelli F, Capasso R, et al. Non-psychotropic plant cannabinoids: new
therapeutic opportunities from an ancient herb. Trends Pharmacol Sci
2009;30:515–27.
39. Massi P, Solinas M, Cinquina V, et al. Cannabidiol as potential anticancer drug.
Br J Clin Pharmacol 2012.
40. Bisogno T, Hanus L, De Petrocellis L, et al. Molecular targets for cannabidiol and
its synthetic analogues: effect on vanilloid VR1 receptors and on the cellular
uptake and enzymatic hydrolysis of anandamide. Br J Pharmacol
2001;134:845–52.
41. Salazar M, Carracedo A, Salanueva IJ, et al. Cannabinoid action induces
autophagy-mediated cell death through stimulation of ER stress in human
glioma cells. J Clin Invest 2009.
42. Carracedo A, Gironella M, Lorente M, et al. Cannabinoids induce apoptosis of
pancreatic tumor cells via endoplasmic reticulum stress-related genes. Cancer
Res 2006;66:6748–55.
43. Vara D, Salazar M, Olea-Herrero N, et al. Anti-tumoral action of cannabinoids on
hepatocellular carcinoma: role of AMPK-dependent activation of autophagy.
Cell Death Differ 2011;18:1099–111.
44. Oesch S, Walter D, Wachtel M, et al. Cannabinoid receptor 1 is a potential drug
target for treatment of translocation-positive rhabdomyosarcoma. Mol Cancer
Ther 2009;8:1838–45.
45. Gustafsson K, Christensson B, Sander B, et al. Cannabinoid receptor-mediated
apoptosis induced by R(+)-methanandamide and Win, 55,212-2 is associated
with ceramide accumulation and p38 activation in mantle cell lymphoma. Mol
Pharmacol 2006;70:1612–20.
46. Massi P, Vaccani A, Bianchessi S, et al. The non-psychoactive cannabidiol
triggers caspase activation and oxidative stress in human glioma cells. Cell Mol
Life Sci 2006;63:2057–66.
47. McKallip RJ, Jia W, Schlomer J, et al. Cannabidiol-induced apoptosis in human
leukemia cells: a novel role of cannabidiol in the regulation of p22phox and
Nox4 expression. Mol Pharmacol 2006;70:897–908.
48. McKallip RJ, Nagarkatti M, Nagarkatti PS. Delta-9-tetrahydrocannabinol
enhances breast cancer growth and metastasis by suppression of the
antitumor immune response. J Immunol 2005;174:3281–9.
49. Zhu LX, Sharma S, Stolina M, et al. Delta-9-tetrahydrocannabinol inhibits
antitumor immunity by a CB2 receptor-mediated, cytokine-dependent
pathway. J Immunol 2000;165:373–80.
50. Gardner B, Zhu LX, Sharma S, et al. Methanandamide increases COX-2
expression and tumor growth in murine lung cancer. FASEB J 2003;17:2157–9.
51. Blazquez C, Carracedo A, Barrado L, et al. Cannabinoid receptors as novel targets
for the treatment of melanoma. FASEB J 2006;20:2633–5.
52. Chan PC, Sills RC, Braun AG, et al. Toxicity and carcinogenicity of delta 9-
tetrahydrocannabinol in Fischer rats and B6C3F1 mice. Fundam Appl Toxicol
1996;30:109–17.
53. Takeda S, Yamamoto I, Watanabe K. Modulation of Delta9-
tetrahydrocannabinol-induced MCF-7 breast cancer cell growth by
cyclooxygenase and aromatase. Toxicology 2009;259:25–32.
54. Takeda S, Yamaori S, Motoya E, et al. Delta(9)-Tetrahydrocannabinol enhances
MCF-7 cell proliferation via cannabinoid receptor-independent signaling.
Toxicology 2008;245:141–6.
55. Sulcova E, Mechoulam R, Fride E. Biphasic effects of anandamide. Pharmacol
Biochem Behav 1998;59:347–52.
56. Calatozzolo C, Salmaggi A, Pollo B, et al. Expression of cannabinoid receptors
and neurotrophins in human gliomas. Neurol Sci 2007;28:304–10.
57. Ellert-Miklaszewska A, Grajkowska W, Gabrusiewicz K, et al. Distinctive
pattern of cannabinoid receptor type II (CB2) expression in adult and
pediatric brain tumors. Brain Res 2007;1137:161–9.
58. Sanchez C, de Ceballos ML, Gomez del Pulgar T, et al. Inhibition of glioma
growth in vivo by selective activation of the CB(2) cannabinoid receptor. Cancer
Res 2001;61:5784–9.
59. Fowler CJ, Hammarsten P, Bergh A. Tumour Cannabinoid CB(1) receptor and
phosphorylated epidermal growth factor receptor expression are additive
prognostic markers for prostate cancer. PLoS One 2010;5:e15205.
60. Gustafsson SB, Palmqvist R, Henriksson ML, et al. High tumour cannabinoid CB1
receptor immunoreactivity negatively impacts disease-specific survival in
stage II microsatellite stable colorectal cancer. PLoS One 2011;6:e23003.
61. Zheng D, Bode AM, Zhao Q, et al. The cannabinoid receptors are required for
ultraviolet-induced inflammation and skin cancer development. Cancer Res
2008;68:3992–8.
62. Wang D, Wang H, Ning W, et al. Loss of cannabinoid receptor 1 accelerates
intestinal tumor growth. Cancer Res 2008;68:6468–76.
63. Carracedo A, Lorente M, Egia A, et al. The stress-regulated protein p8 mediates
cannabinoid-induced apoptosis of tumor cells. Cancer Cell 2006;9:301–12.
64. Galve-Roperh I, Sanchez C, Cortes ML, et al. Anti-tumoral action of
cannabinoids: involvement of sustained ceramide accumulation and
extracellular signal-regulated kinase activation. Nat Med 2000;6:313–9.
65. Casanova ML, Blazquez C, Martinez-Palacio J, et al. Inhibition of skin tumor
growth and angiogenesis in vivo by activation of cannabinoid receptors. J Clin
Invest 2003;111:43–50.
66. Grotenhermen F. The toxicology of cannabis and cannabis prohibition. Chem
Biodivers 2007;4:1744–69.
67. Portenoy RK, Ganae-Motan ED, Allende S, et al. Nabiximols for opioid-treated
cancer patients with poorly-controlled chronic pain: a randomized, placebo-
controlled, graded-dose trial. J Pain 2012.
68. Wade DT, Collin C, Stott C, et al. Meta-analysis of the efficacy and safety of
Sativex (nabiximols), on spasticity in people with multiple sclerosis. Mult Scler
2010;16:707–14.
69. Guzman M. Cannabinoids: potential anticancer agents. Nat Rev Cancer
2003;3:745–55.
70. Robson P. Abuse potential and psychoactive effects of delta-9-
tetrahydrocannabinol and cannabidiol oromucosal spray (Sativex), a new
cannabinoid medicine. Expert Opin Drug Saf 2011;10:675–85.
71. Torres S, Lorente M, Rodriguez-Fornes F, et al. A combined preclinical therapy
of cannabinoids and temozolomide against glioma. Mol Cancer Ther
2011;10:90–103.
72. Donadelli M, Dando I, Zaniboni T, et al. Gemcitabine/cannabinoid combination
triggers autophagy in pancreatic cancer cells through a ROS-mediated
mechanism. Cell Death Dis 2011;2:e152
73. Miyato H, Kitayama J, Yamashita H, et al. Pharmacological synergism between
cannabinoids and paclitaxel in gastric cancer cell lines. J Surg Res
2009;155:40–7.
74. Gustafsson SB, Lindgren T, Jonsson M, et al. Cannabinoid receptor-independent
cytotoxic effects of cannabinoids in human colorectal carcinoma cells: synergism with 5-fluorouracil. Cancer Chemother Pharmacol 2009;63:
691–701.
75. Marcu JP, Christian RT, Lau D, et al. Cannabidiol enhances the inhibitory effects
of delta9-tetrahydrocannabinol on human glioblastoma cell proliferation and
survival. Mol Cancer Ther 2010;9:180–9.